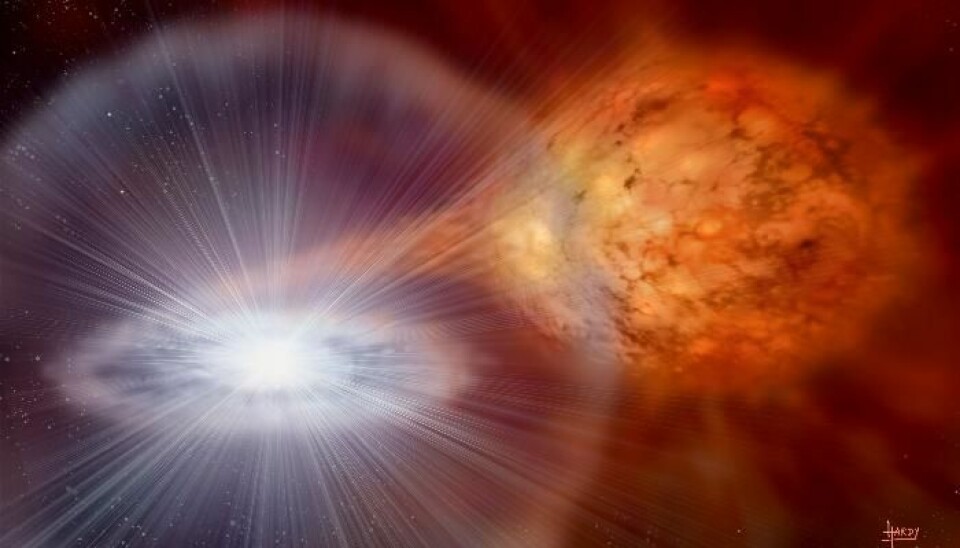
NICER will reveal the mysteries of neutron stars
Did you know that neutron stars have many of the same properties as black holes? The big difference being that we can actually see them. A new NASA-mission has just set out to explore the many mysteries of neutron stars.
Astrophysicists have studied neutron stars with many different techniques since 1967.
Neutron stars were first theorised in 1934 as the remnant cores of stars about 10-20 times more massive than the Sun, after they have exploded in supernovae. Despite their name, neutron stars are not the same as the gaseous fireballs that we are used to seeing in the night sky, such as our Sun.
Neutron stars are in fact very solid and compact, made of the hardest matter observed in the Universe. They compress about twice the mass of the Sun inside a sphere of approximately 20 km diameter, about the size of a city like Copenhagen.
Therefore their density is similar to the nuclear density, which is equivalent to one billion tons per cubic centimetre, or the weight of all people that have ever lived on Earth, squeezed onto a teaspoon!
The gravity on the surface of such a compact object is one hundred billions times the gravity we feel on Earth, leading to an escape velocity about half the speed of light. This means that a neutron star is almost a black hole, for which the escape velocity is by definition equal to the speed of light. The magnetic field of neutron stars is typically also about one hundred billions times the magnetic field of the Earth. Finally, many neutron stars spin very fast, up to several hundred rotations per second.
This all fits with what we ‘think’ we know about neutron stars. However, there are still many unanswered questions regarding these mysterious objects.
It is these mysteries that the ‘Neutron star Interior Composition ExploreR’ (NICER) will address.
NICER is developed by NASA, with help from scientists at DTU Space. It is the first instrument specifically dedicated to observe neutron stars and is now operational aboard the International Space Station.
Two classical ways to observe neutron stars
Neutron stars can be observed in different incarnations: the most common are pulsars, the first neutron stars discovered with radio telescopes, 50 years ago.
These are isolated neutron stars that produce electromagnetic emission through their strong magnetic field in rapid rotation like a dynamo. Each time the beam of radiation points towards the direction of Earth we detect a short radio pulse, which is where the name ‘pulsar’ comes from.
However, many other neutron stars are most easily observed in X-rays, especially when they gravitationally attract matter from an orbiting companion star in a binary system. In such a case, the stellar plasma, before falling onto the surface of the neutron star, is amassed around it, forming an accretion disk as a kind of reservoir where the matter is warmed up by friction and reaches temperatures of millions degrees.
Now, try to imagine these very compact objects like huge magnets as large as the city of Copenhagen, that are spinning faster than the drum of a washing machine. You would actually not be able to see a neutron star spin unless you have the right instrument, such as a high-speed camera with a high enough timing resolution--the ability to make a measurement with high precision during a very short time. This is the equivalent of taking pictures of a bee’s wings in motion.
Collaboration between NASA and DTU
High timing resolution is exactly what the new NASA mission NICER provides, as well as high spectral energy resolution, meaning that the energy of each parcel of light (photon) coming from the neutron stars can be measured with high precision.
NICER was launched on 3rd June 2017 with a SpaceX Falcon-9 rocket from Cape Canaveral in Florida. It was installed and deployed a few days later on the International Space Station (ISS) orbiting at about 400 km above Earth.
NICER consists of an array of 56 co-aligned X-ray concentrators (gold-coated mirrors that converge the X-ray light, but do not focus it as a telescope would) and the same number of silicon-drift detectors.
Thanks to this advanced technology, NICER is achieving much better performance than any other X-ray instrument already used to observe neutron stars.
DTU Space designed, built, and delivered the star-tracker system that enables NICER to be permanently orientated towards the targets of the X-ray observations with very high accuracy. This revolutionary pointing system compensates for the orbital motions and vibrations (for example due to the astronaut activities) of the ISS, which could otherwise add noise to the data and affect the precision of the timing measurements of pulsars. Beyond this technological collaboration, DTU Space also participates in the overall research program and is engaged in the scientific analysis of the data obtained by NICER.
NICER aims to determine the state of matter
With this new instrument, we will be able to better quantify the actual size of neutron stars.
While the mass of some neutron stars has been pretty well measured thanks to observations of double neutron star systems and the use of general relativity, their dimension is not as well known. For example, are neutron stars actually as large as a city like New York or are they more like Oslo?
One of the main purposes of the NICER mission is to determine the physical state of matter when compressed into the extreme high densities that exist in the core of neutron stars, which cannot be reproduced in any laboratory on Earth.
In fact, we do not know exactly what these compact objects we call neutron stars are really made of.
The structure of the neutrons is likely deformed into thin plates and elongated tubes. And it may even turn out that neutron stars are actually not (only) made of neutrons, but (also) of more exotic sub-nuclear particles like pions or quarks.
Watch the deployment of NICER and learn more about how it will help scientists from NASA and DTU to understand neuron stars.(Video: NASA)
The difference between a black hole and a neutron star
As mentioned above, what differentiates neutron stars from black holes is that light can escape from the surface of a neutron star, which means that unlike black holes, we can directly observe them.
So, whereas it is practically impossible to know anything about black holes apart from their mass, we do know that they affect their environment in a similar way to neutron stars. So it can be difficult to tell them apart.
The best way to identify a neutron star, is by observing phenomena originating from their surface, which can be used to state that they are definitely not black holes.
For example, the large mass of neutron stars compressed into a small volume (i.e., their compactness) creates a huge gravitational field that is able to curve space-time, just like a black hole, though to a lesser degree. The light rays follow this curvature making it possible to see the far side of the neutron stars, while in a black hole the light path is curved such that it falls back into the hole.
A better understanding of what goes on inside a neutron star
NICER will use this behaviour to measure the compactness (i.e. the ratio of its mass to its radius) of pulsars. Specifically, it will follow their rotation by investigating signal modulations arising from light bending caused by general relativity effects.
The higher the compactness, the more we can see of the entire surface of the neutron star , which has the effect to decrease the amplitude of the light variation when the star rotates.
We also want to understand how the huge energy of neutron stars is radiated through pair-particle production and ultra-relativistic acceleration within their magnetosphere.
As other goals, NICER will also investigate the neutron stars’ dynamic phenomena like glitches and X-ray bursts. The former are observed as sudden accelerations of the spin rate and may be caused by superfluid whirlpools thought to develop in the interior of the neutron stars. The latter are thermonuclear explosions on the neutron stars’ surface that we can observe as sudden flashes of X-rays.
Depending on the actual amount of the accreted stellar matter that is consumed during the thermonuclear X-ray bursts, they may last from seconds to hours. Thanks to the enhanced capabilities of NICER, it will for the first time be possible to identify some heavy elements that are produced as ashes of these titanic explosions whose energy release is of the order of one hundred billions of billions of times the Hiroshima atomic bomb.
This will allow us to much better understand the thermonuclear reactions that also take place in the core of stars, but that we cannot observe directly. If some of the elements synthesised during X-ray bursts are ejected in the cosmos with the wind of the explosions, it is in principle possible that some of the atoms in our bodies were forged on neutron stars.