Researchers' Zone:

Living human brain in petri dishes provides unique insight into old mysteries
For the first time in Denmark, researchers are examining living pieces of human brain in a laboratory.
Your brain is on 24/7, it is what allows you to learn a new language, plan out your day, remember a happy memory and even what allows you to dream. But the question of exactly how it all works is still a mystery to us.
Neuroscience often looks to animal models for answers. However using the mouse brain to understand the human brain is not a perfect solution.
Researchers compare this to using an old flip phone as a model to learn to fix todays smartphone. Sure, they have some conserved features, such as calling and storing contacts, but the smartphone is so much more complex.
That is why, in our lab at the department of biomedicine at Aarhus University, we go straight to the source, we test our hypotheses directly in human tissue. As a first in Denmark, we keep human brain tissue alive for two weeks.
This is no small undertaking, it takes a lot of coordination. Denmark has encouraged researchers and clinicians to work together and in Aarhus in particular, we have a strong connection with the hospital, allowing for clinicians to work directly with researchers.
We work with neurosurgeons from Aarhus University Hospital, who recruit patients already scheduled to undergo a craniotomy for medical reasons to donate tissue to the project.
We are then informed of when the surgery will be and what area the brain tissue will be taken from. It could be frontal, parietal or temporal cortex, areas of the brain important for higher-order thinking.
Sometimes even tissue from the cerebellum, an area important for movement and motor control.
Preparing for surgery
The day before surgery, the lab is busy with preparation. We need to prepare solutions that made up of the same ions and minerals as the cerebral spinal fluid that your brain is floating in. We call this artificial cerebral spinal fluid or aCSF.
The day of surgery usually starts early, at 7 am to finish the last of the preparation. We arrive at the hospital around 9:30.
Sometimes we have time to grab a coffee, other times, it’s straight into the change room to put on scrubs and go into the surgery.
It is not very often that researchers get to see what happens on the clinical side, so it is really exciting as a researcher to see the surgeons in action.
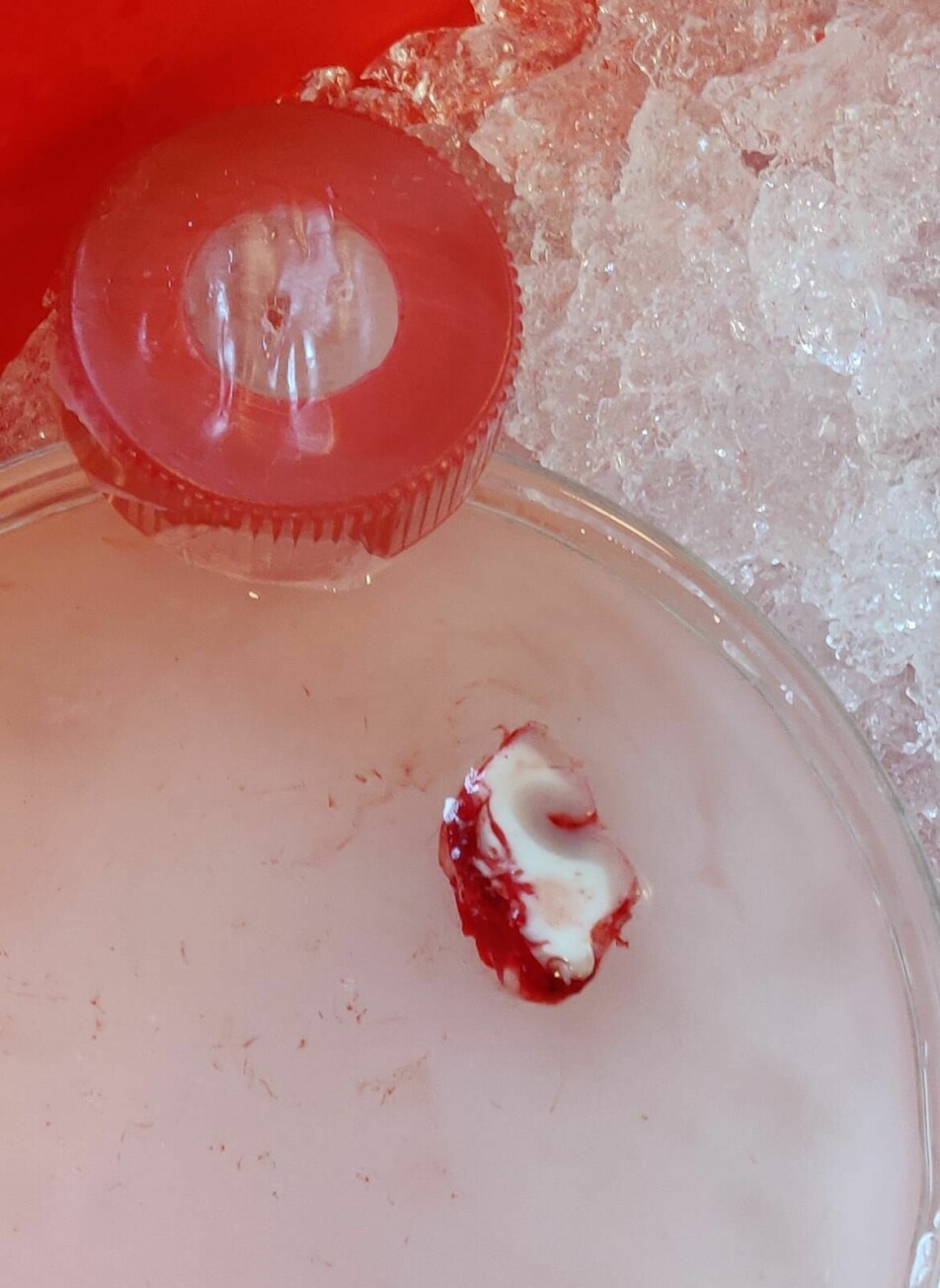
In the surgery room, the surgeon carefully extracts a piece of brain tissue approximately 1cm3. We are there with our tube of aCSF for the brain to be placed directly into.
Now the clock has started. Once the brain is outside of the skull, blood vessels are cut off, neurons, the brains central building blocks, without oxygen delivered via blood vessels, begin to die.
The processes for cell death are activated, so our first step is to cool down the tissue to slow this process and keep it oxygenated to keep cells alive.
We do this by keeping out tube in a cooled thermos and carrying a small gas tank with us. We then drive back to the university with our sample to begin our experiments.
In the lab
We can’t use the tissue sample as it is. We first need to slice it into thin, 350 µm slices (approximately one third of a millimeter).
This way we have many smaller samples to work with, and the sections are thin enough that we can visualize the neurons with a microscope.
We then store these slices at room temperature and let them rest for an hour. They have gone through a lot, and deserve a break.
Then we put them in specialized cell culture plates with cell culture media, another solution to keep neurons alive, and place them in an incubator. Incubators are warm and humid, ideal for keeping cells alive.
However, warm and humid may also remind you of bacteria and molds favourite place to grow.
This is why one of the most challenging aspects of our work is keeping everything sterile from the moment we collect the tissue at the hospital to putting it in the incubator.
For the next two weeks, I use a technique called whole-cell electrophysiology, where I record the electrical signals from individual neurons.
For example, I can record the signal from two neurons that are connected, this connection is called a synapse.
Here I can stimulate one neuron and see the reaction in the second neuron. If I repeatedly stimulate the connection between these two neurons, we will see an increase in the strength of that connection.
This is thought to be the cellular basis for learning and the strengthening of connections is called synaptic plasticity. I can also add a drug during this repeated stimulation and see if that strengthens or weakens the plasticity.
I typically spend 6 hours recording the electrical signals of neurons from a single brain slice.
Neural mechanisms of learning and memory
Our research focusses on how neurons communicate with eachother. By understanding how neurons communicate, and how we can strengthen that connection we hope to understand more about the mechanisms behind learning, memory and attention.
We recently published a study about how the neurotransmitter dopamine impacts neuronal communication and the differences between human and mouse neurons.
We found that both the adult human and mouse neurons are less plastic than neurons from younger brains, meaning the connections do not strengthen as easily.
We also see this in humans, as it is more difficult for the brain to regain functions when connections are lost. For example in the case of a blood clot in the brain, speech and motor functions are often lost. And it has been shown that children and young adults recover much more function than the elderly.
We also applied dopamine during our experiments. Dopamine is a molecule made in the brain that you may have heard about being associated with rewards such as chocolate or likes on social media.
We found that dopamine strengthened the connections between neurons, however, the mechanisms behind this strengthening appeared to be different in the human versus the mouse neurons.
Your neurons are covered in small receptors for all kinds of signaling molecules. These receptors wait around until the right molecule comes along and binds with it, like two pieces of a jigsaw puzzle fitting together, to pass on the signal.
The molecule, in our case dopamine, is released from one neuron and binds to the dopamine receptors found on the surface of another neuron.
However, a massive undertaking from the Allen Institute for Brain Science has mapped out the expression of different receptors in the brain, and while some receptors are expressed in a similar pattern in the human and mouse brain, many are not, including dopamine.
This can mean that different species will have vastly different responses to drugs as these also bind to receptors in order to work.
We believe the differences seen in our study could be due to differences in how dopamine receptors are expressed in different species.
More research directly in human tissue
This brings us back to the question of why science keeps using animal models. Why we keep using the flip phone to understand the smartphone.
There is a level of specificity available with animals models that we could not replicate in the human tissue.
With genetically modified animals we can selectively label a specific neuron type. Combine that with viral tools such as optogenetics, where a light can be used to activate a neuron instead of a drug or electric current, there is a level of specificity we have not been able to achieve in the human tissue.
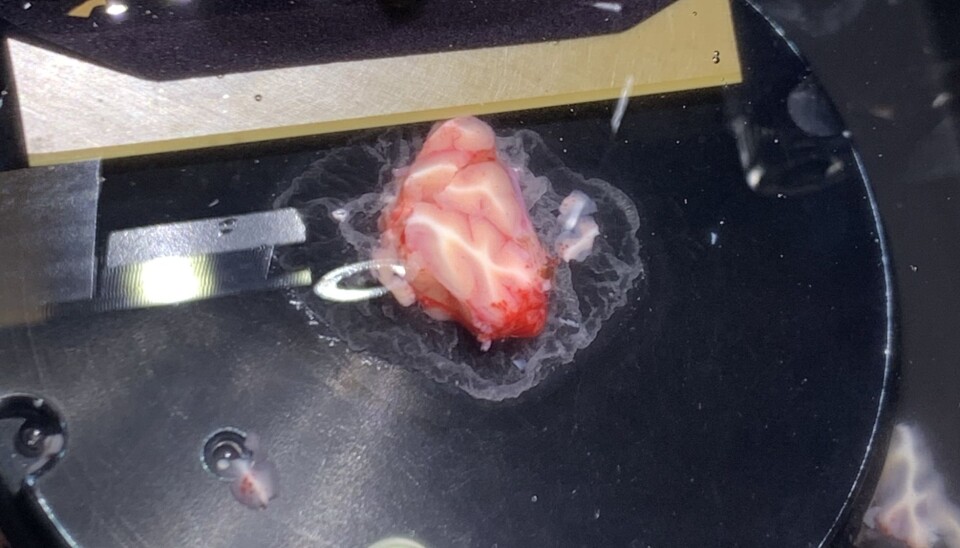
We can activate a specific population of neurons in a specific area in a mouse, whereas with the human tissue, we could not do this.
In the past, when we received human tissue, we could only keep it alive for about 24 hours.
Therefore, these limitations made the human brain tissue experiments very labour intensive and you couldn’t get the quantity or quality of experiments you could get from an animal model.
In our study, it was much quicker to acquire the mouse data and we were able to dig deeper into the mechanisms behind dopamine’s actions in the mouse, in ways we couldn’t in the human tissue.
Now that we are able to culture that tissue for two weeks we can get much more data. But the most recent advancement has been in fast-acting viruses targeting specific types of human neurons.
We are now able to label specific neuron types in the human tissue. Meaning that our research is approaching the level of specificity of animal research, making this approach a good platform for translational research.
Do you have someones memories in there?
Of course, when taking a piece of a persons brain, the ethical implications must be considered. All of our work is reviewed and approved by an ethics committee. Three common questions we are asked:
1. Can it feel pain? The short answer is no. the brain doesn’t have nocioceptors (pain receptors) and therefore doesn’t feel anything. This is also why doctors can do some open brain surgeries on awake patients.
2. Is it conscious? Could you extract someones memories? While we don’t know the exact neural correlates of consciousness, we can say that higher-order cognitive thinking (decision making, planning, attention etc) requires many brain regions and they need to be able to communicate with eachother. As well, memories are believed to be stored through synaptic connections and not in individual cells. In short, you would need way more tissue than our tiny brain cube to come anywhere near consciousness or memories.
3. Why would people consent to this? These patients are undergoing the craniotomy due to a brain tumour that needs to be removed. We do require patient consent to take the tissue and the doctor explains that the tissue will be used for research. However, the piece we receive is part of the still healthy cortex away from the tumour that will be removed anyway. And our procedure only lengthens the already mutlihour surgery by about one minute. So patients have been extremely generous and almost everyone agrees to donate.
Here is Aarhus, we are extremely fortunate to have this unique experimental setup, with generous patients, scientifically curious neurosurgeons and persistent researchers.
Emma Louth conducted this research in the laboratory of Marco Capogna at Aarhus University, Department of Biomedicine, in collaboration with Jens Christian Hedemann Sørensen and Anders Rosendal Korshøj, at Aarhus University Hospital, Department of Neurosurgery.
Read the Danish version at our sistersite Forskerzonen.
References:
Emma Louise Louth's profile (Aarhus Universitet)
Emma Louise Louth's profile (University of Copenhagen)